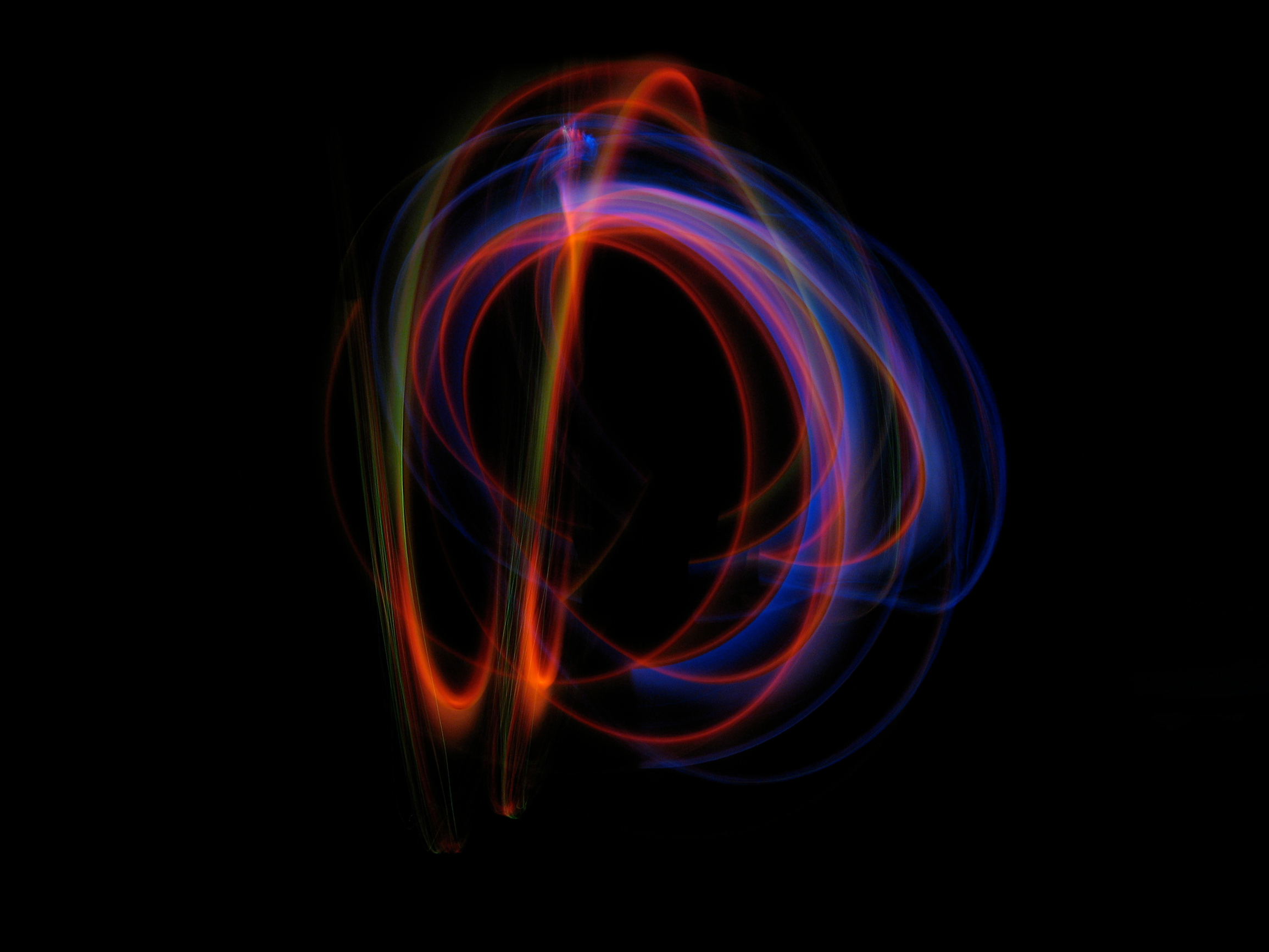
The default chassis of expression for a technically demanding product that requires bioprocessing manufacture tends to be Chinese Hamster Ovary (CHO) cells, E.coli or forms of yeast such as Pichia pastoris and Saccharomyces cerevisiae, usually from a cassette on a plasmid. In recent years, synthetic biology has begun to offer improvements of the same microbes and cassettes, powered by in-silico methods of adapting and exploring large numbers of suitable scenarios. Yet all these options have scientific limitations that only a new approach harnessing the power of evolution can resolve.
Challenges of bioprocessing
Bioprocessing manufacture usually involves a host cell that generates a molecule/chemical or protein, the latter being either secreted out of the cell or within the cell, after which it is burst open for recovery. The product specification needs to be encoded by a gene or genes (in the case of enzymes leading to a molecule/chemical) in the host cell. For a protein the gene sequence for the product is inserted into the host cell using a plasmid, and this determines the nature of the product secreted or generated.
Frequently however it is only possible to create something close to the desired protein, peptide or other product. The product generated by the host cell then has to be adjusted or purified in downstream processing steps tailored to its specific requirements. Purification may also be needed to eliminate proteins from the host cell that linger in the final product. The level of purification will depend on the product’s intended use and the regulations that apply.
The nature of the host cell also has an impact. The proteins of certain hosts such as E.Coli are highly toxic to humans. This creates greater demands on purification and escalates the associated risk.
Adjusting the genome
Adjusting the genome of the plasmid genome and the host cell genome plays a part in most projects that require bioprocessing manufacture. The plasmid genome is easily manipulated and this is where synthetic biology is usually employed. The host genome is usually only tweaked by single steps, whether by rational engineering based on previous experience, or by mutagenesis. Manufacturing the correct specification consistently at scale is a major challenge. So is achieving the necessary titre of production, and making upstream adjustments to reduce downstream processing and purification requirements. In the world of bioprocessing, these steps determine whether an idea is transformed into a commercially and technically viable product – or not.
Limitations of traditional genome chassis
Contract development and manufacturing organisations (CDMOs) tend to use genome chassis that are tried and tested, with the genome engineered to match the requirements of the product. This can work well for relatively uncomplicated jobs such as mainstream monoclonal antibodies in CHO, but less so for more technically or commercially challenging projects.
That is partly because a standard chassis is traditionally a fixed genome combination based on whatever has worked before. But every genome is a combination of different variants of alleles for each gene, and so this traditional approach – which is dependent on the experience, skillset and bias of the vendor – ignores the huge potential of using different combinations of allelic variants.
Unsurprisingly CDMOs do not tend to highlight the limitations of genome engineering; nor do companies that sell synthetic biology, AI and machine learning technologies in this field. However, for a customer with a product requirement, committing a fixed payment to a CDMO with no guarantee of achieving the desired specification within a reliable timeframe, is a considerable risk. Just because CHO has previously delivered monoclonal antibodies does not mean it will meet the specification of a different protein or material at the necessary titre.
Harnessing the power of evolution
For harder or commercially more ambitious projects, new methods are needed to harness the power of evolution and access more of the genomic spectrum. Evolution is the result of natural selection testing the variation of genes in the context of the whole genome. Robust yeast strains will each have a combination of genetic variation across the genome that has appropriate phenotypes for its environment. Recombining these variants into new combinations results in new phenotypes. Inevitably, some are maladapted while others are better than in the original strains. The interactions across multiple variants provide new and unpredictable phenotypic outcomes that hold significant potential for optimisation of the specific bioprocess in question.
Quantitative trait loci technology
New QTL technology uses libraries of genotypes that provide potentially infinite numbers of phenotypes for the creation of new products. Evolution has provided variants that pass the test of natural selection in their parent strain context. Our breeding recombines these, and those combinations that are alive have passed nature’s test. We can generally screen a billion different unique strains for improved phenotype as desired, and can successively breed from the best for further improvements. Through breeding, screening and proprietary analysis of the key traits of the genome, successive rounds can optimise your strain to the needs of your product.