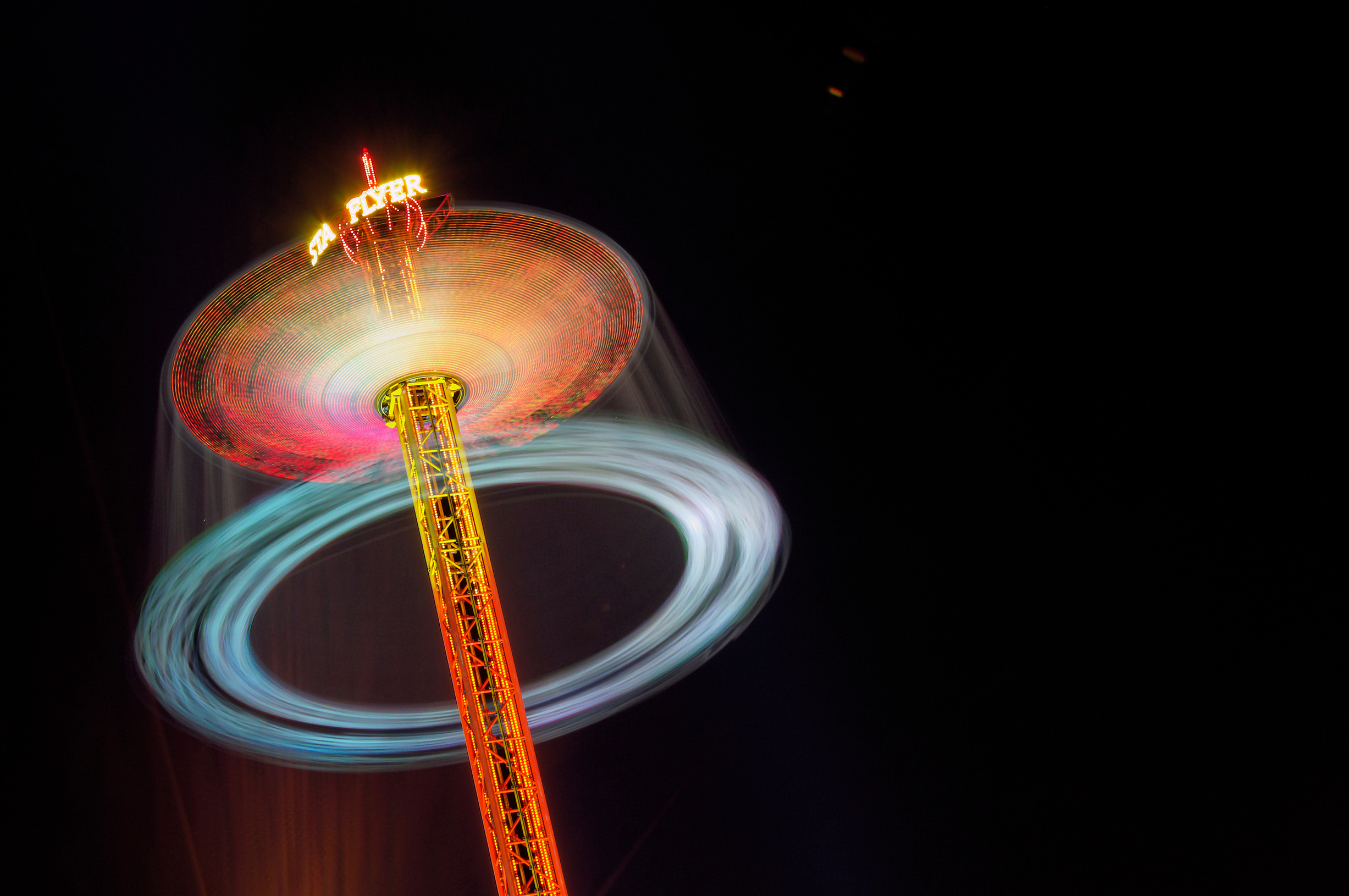
Human serum albumin (HSA) was first produced from blood donations using the Cohn fractionation process during World War II. This method involves precipitating different plasma proteins at various ethanol concentrations, pH, and temperatures. Albumin is usually obtained in Fraction V of the Cohn process.
Pathogen fears
In the 1980s, work began to produce recombinant HSA, including from baker’s yeast, Saccharomyces cerevisiae, primarily to address concerns about emerging blood-borne viral pathogens such as HIV. A variety of other expression systems were also explored for albumin production, including the yeast, Pichia pastoris and rice (Oryza sativa), which, like baker’s yeast, are used for commercial manufacture of different grades of rHSA today (Chen et al., 2013).
Commercially available albumins from human plasma, rice, Pichia, and baker’s yeast all differ in their post-translational modifications (PTMs), the ligands bound to them, and their purity in relation to host proteins and other process-related impurities. Selecting the correct rHSA product depends on the intended use.
Post-translational modifications matter
Some applications require albumin molecules that do not contain significant levels of certain non-human post-translational modifications. Examples are where albumin enters the bloodstream in vaccine formulations such as MMR-II®, or when albumin is used as an excipient in therapeutic biologics or cell therapies. These product-related impurities might cause undesirable side effects, including adverse immunogenic responses in humans. Such PTMs may also impair the ability of the modified albumin to function correctly when binding ligands and receptors.
Manufacturing risks
Some recombinant albumins, especially from rice and Pichia pastoris, have been shown to contain glycosylation likely to trigger immune responses, which included non-enzymatic glycosylation of lysine and arginine side chains. High titers of antibodies directed primarily to the mannan component of Pichia pastoris components have been found in normal human serum because of the similarity in structure between Candida albicans proteins that may precipitate cross-reactivity (Chuang et al., 2007). The elimination of such a component is, therefore, critical for product safety. While albumin from Pichia pastoris was commercially launched in 2007-2008 (marketed as Albrec and Medway), compliant manufacturing issues subsequently resulted in the product being withdrawn from the market in 2009.
Metal binding
Albumin also has an important metal binding site at its N-terminus for copper, zinc, nickel, and other metals. However, this site will not function in all commercially available albumins, especially those from Pichia pastoris that lack the N-terminal aspartic acid residue. There is also a natural microheterogeneity in plasma-derived HSA due to the loss of the N-terminal Asp (Aspartate) and Asp-Ala (Aspartate-Alanine) residues over time, which will similarly prevent metal binding at this site.
For retaining the antioxidant properties of albumin, high levels of free-thiol at cysteine-34 are needed. This also matters if the albumin is being used for drug conjugation to cysteine-34. Amongst the recombinant albumins, rHA from baker’s yeast, Saccharomyces cerevisiae, typically has consistently high levels of free-thiol combined with negligible risk of adverse immune reactions from PTMs.
Know your ligands
Due to the wide variety of possible albumin-binding ligands, commercially available albumins tend to carry different ligands based on their different origins, purification and formulation processes. For plasma-derived HSA, differences in donor albumin can contribute significantly to batch-to-batch variations. Ligand differences can arise from different expression hosts for recombinant albumins, as well as differences in purification and formulation. Like the post-translational modifications described above, the different ligands on commercially available albumins can affect their suitability for certain applications, especially for regenerative medicine.
Assessing albumin purity
In addition to the albumin molecules and their ligands, commercially available albumins contain different process-related impurities. For plasma-derived albumins, donor screening for known pathogens combined with viral reduction during the modernised Cohn process – for example, from ethanol precipitation, heat treatment, and filtration – can reduce the risk of viral and prion pathogens. However, while these measures may reduce the overall risk of viral and prion contamination, the possibility cannot be completely eliminated. This applies to emerging viral pathogens in particular, some of which are currently unknown. Regulatory authorities therefore advise replacing animal-derived albumin with recombinant albumin whenever a suitable replacement is available.
A safe and reliable source
Fortunately, it is possible to manufacture recombinant albumins with exceptionally low levels of process-related impurities, which can also be halal-compliant and completely free of animal-derived components. Consistent high-quality, fully functional albumin without any post-translational modification issues was first produced from baker’s yeast, Saccharomyces cerevisiae, which was successfully tested in Phase I trials, including up to 50 gram intravenous doses, as described by Bosse et al., 2005. Recombinant albumin from baker’s yeast has also been used to define the United States Pharmacopeia (USP) monograph reference standard recombinant albumin. Baker’s yeast still provides the safest and most reliable source of recombinant albumin today.